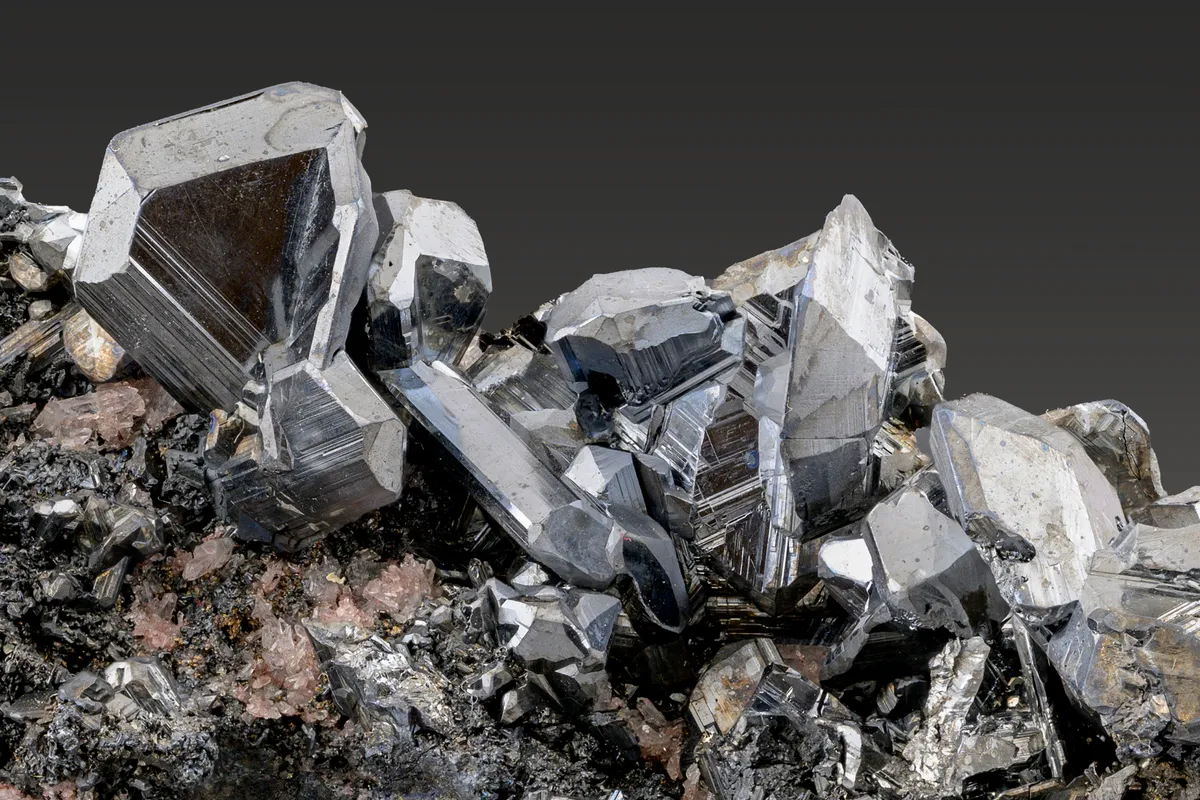
Image Credit: Malcolm Southwood
Title
chalcocite
Composition
Cu2S
Crystal System
Monoclinic
Status at Tsumeb
Confirmed
Abundance
Very common
Distribution
First, second and third oxidation zones; sulphide ores.
Paragenesis
Hypogene (?); supergene.
Type Locality
No
Entry Type
Species TSNB76
General Notes
Chalcocite is an important ore mineral throughout the Tsumeb deposit.
Klein (1938) observed the distribution of chalcocite between the surface and 22 Level (the maximum depth reached at that time). He noted that chalcocite is the most common copper sulphide, occurring at all levels but with varying habits. Massive chalcocite was common in the upper levels, giving way to "spongy" chalcocite that persisted to a depth of 400 meters (14 Level). Klein also noted the occurrence of "blue chalcocite" (an obsolete name for digenite), between 420 and 460 meters (i.e. down to 16 Level).
Lombaard et al. (1986) noted the importance of chalcocite throughout the deposit, but in amounts that varied with depth. Between the surface and 12 Level, chalcocite accounted for typically 10-15% of the ore by volume, increasing to more than 20% by volume between 12 and 16 levels before dwindling to less than 5% below 24 Level. The volume of chalcocite picks up again in the second oxidation zone (c. 15% by volume on 30 Level), but then tails off to around 4% below 38 Level.
Solid masses of chalcocite to 20 cubic metres occurred in the first oxidation zone (Keller, 1977). Acicular crystals (an unusual habit for this species) were found in the second oxidation zone. The South 95 manto ore body, also in the second oxidation zone, consisted largely of chalcocite (Lombaard et al. 1986). Well-formed crystals (to 40 mm) were found in the third oxidation zone, between 44 and 47 levels, some of them associated with stolzite and nickel-bearing siderite (Gebhard, 1999).
Chalcocite occurs mainly as a component of massive sulphide ores, both hypogene and supergene, with the latter predominant.
Well-formed, free-standing crystals of chalcocite are comparatively rare and highly prized by collectors; almost certainly, these are all of supergene origin. They occur in a diverse range of habits from tabular, through prismatic, to acicular. Twinning on {011} – the so-called V-twins, which commonly repeat to form pseudo-hexagonal plates – is common. It is possible that the acicular habit of chalcocite can be confused with betekhtinite, furutobeite or stromeyerite, although these minerals are very much rarer.
Most, if not all "chalcocite" at Tsumeb is an intimate mixture of at least two different species - chalcocite and djurleite; less commonly, digenite and roxbyite (Blass et al. 2009) and possibly anilite (Hughes 1987) may also be present. In many cases, these minerals cannot be reliably distinguished visually, even by ore microscopy. According to Gebhard (1999), however, X-ray studies showed crystals recovered from the third oxidation zone, between levels 44 and 47, comprise a more-or-less equal mix of chalcocite and djurleite.
Most authors agree on the importance and abundance of supergene chalcocite at Tsumeb, but there has been some debate as to how much of the chalcocite, particularly in the "unoxidised" ores between the oxidation zones, is of hypogene origin.
Pioneering ore microscopy studies by Schneiderhöhn (1920a,1920b, 1929 for example) recognised "granular chalcocite" from between 2 and 5 levels. This chalcocite replaces pyrite and bornite and was interpreted by Schneiderhöhn as supergene in origin. Below 5 Level, Schneiderhöhn described "lamellar" chalcocite as predominant, and noted that it exhibited textures indicative of inversion from a higher temperature form, and rounded inclusions of bornite. He interpreted "lamellar" chalcocite as a hypogene phase.
"Lamellar" chalcocite also occurs in ores from deeper in the mine, well below the first oxidation zone (Strunz and Tennyson 1967) and has sometimes been interpreted or assumed to be of hypogene origin.
Geier (1962) distinguished between hypogene chalcocite and "sooty" supergene chalcocite, without commenting on their distribution or relative abundance.
Haynes (1984/1985) noted the occurrence of bornite-chalcocite exsolution textures in primary ores from 16 and 30 levels. Notwithstanding this limited sample base, he suggested the following paragenetic sequence for the hypogene sulphides based on ore microscopy and thermochemical modelling:
chalcopyrite >> bornite >> chalcocite >> enargite >> tennantite
Lombaard et al. (1986) listed chalcocite as a principal hypogene mineral but, curiously, they omitted it from their paragenetic diagram (p.1781). They classify the hypogene ores at Tsumeb as (1) massive peripheral ores; (2) manto ores; and (3) disseminated and stringer ores, with chalcocite listed as a principal component in all three types. Indeed, one ore body – the South 95 manto – was described as "mainly chalcocite". However, they also noted the importance of supergene chalcocite (along with djurleite, digenite and covellite) in both the upper and lower oxidation zones:
"Supergene deposition of chalcocite, digenite, djurleite, and covellite extended down to at least 16 Level [i.e. well below the lower limit of the first oxidation one]. Secondary chalcocite and djurleite are again important ore constituents in the lower oxidation zone."
Hughes (1987) made a compelling case that most of the chalcocite at Tsumeb is supergene. He observed that even in the deeper "unoxidised" ores, chalcocite replaces other sulphide minerals, but that it does not exhibit the deformation textures exhibited by those earlier minerals. He concluded that the chalcocite is post-deformation and therefore of later, supergene origin. According to Hughes, earlier authors missed (or misunderstood) the importance of these deformation fabrics. He considered that supergene sulphide zones at Tsumeb extend to "great depth", well beyond the oxidation zones as we normally define them, and that most of the chalcocite in supposedly "unoxidised" sulphide ore is, in fact, of supergene origin.
Associated Minerals
abellaite; acanthite; adamite; anglesite; anhydrite; anilite (?); argutite; arsenbrackebuschite; arsendescloizite; azurite; baryte; bayldonite; betekhtinite; betpakdalite-CaCa; beudantite; bianchite (?); bornite; brindleyite; brunogeierite; calcioandyrobertsite; calcite; calvertite; chalcopyrite; chlorargyrite; chudobaite; covellite; cuprite; digenite; djurleite; dzhalindite; edwardsite; ekatite; enargite; fahleite; ferberite; fraipontite; gaitite; galena; gallite; gallobeudantite; galloplumbogummite; germanite; germanocolusite; gunningite; gypsum; hemimorphite; hydrozincite; jeanbandyite; johillerite; karibibite; karlseifertite; kësterite; keyite; koritnigite; krieselite; ktenasite; langite; lauraniite; lazaridisite; leightonite; leiteite; libethenite; ludlockite; lukrahnite; malachite; mathewrogersite; mawsonite; nadorite; naumannite (?); niedermayrite; o'danielite; olivenite; otjisumeite; ovamboite; paradamite; posnjakite; powellite; prosperite; pyrite; reinerite; renierite; rhodochrosite (?); roxbyite; schaurteite; scheelite; schneiderhöhnite; siderite; smithsonite; silver; söhngeite; sphalerite; stannite; stolzite; stottite; stranskiite; stromeyerite; sulvanite; tangeite (?); tennantite-(Cu); tennantite-(Zn); thénardite (?); tsumgallite; tungstenite; velikite (?); wurtzite; zincgartrellite; zincolivenite
Pseudomorphs
Chalcocite is reported to form pseudomorphs after the following minerals: enargite (rare); tennantite (rare). Ore microscopy studies have shown chalcocite replacing bornite, galena, and pyrite with the shapes of the precursor minerals sometimes preserved (Hughes, 1987).
The following minerals are reported to form pseudomorphs after chalcocite: betekhtinite (rare); conichalcite (rare); ludlockite (rare).